Enhanced Flux Linkage
Results
and Conclusions
The
figures below show the results of experiments 1 through 6. Note
the difference in orientation between the axes for velocity and
efficiency surfaces.
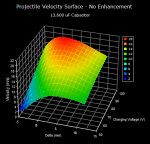
Fig
1a. No Enhancement |
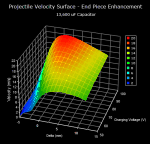
Fig
1b. End Piece Enhancement |
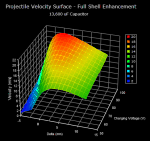
Fig
1c. Full Shell Enhancement |
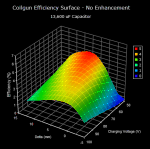
Fig
2a. No Enhancement |
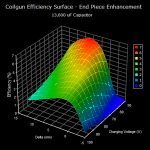
Fig
2b. End Piece Enhancement |
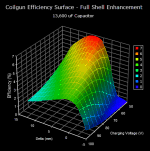
Fig
2c. Full Shell Enhancement |
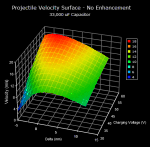
Fig
3a. No Enhancement |
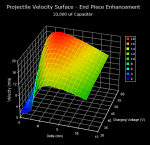
Fig
3b. End Piece Enhancement |
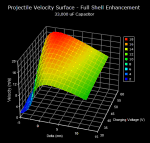
Fig
3c. Full Shell Enhancement |
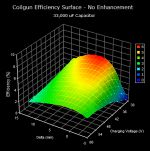
Fig
4a. No Enhancement
|
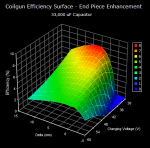
Fig
4b. End Piece Enhancement |
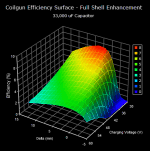
Fig
4c. Full Shell Enhancement |
Effect
of flux enhancement on velocity.
The graphs in figs 5a and 5b show the velocity curves developed
using the 33,000 uF capacitor for charging voltages of 30 V and
60 V respectively.
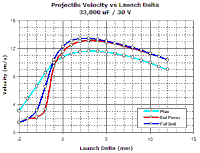
Fig
5a. Various levels of enhancement with a charging voltage
of 30 V.
|
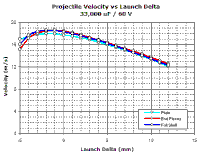
Fig
5b. Various levels of enhancement with a charging voltage
of 60 V.
|
The
introduction of flux enhancement makes a significant difference
to the peak velocity when the charging voltage is 30 V, but there
is very little benefit when the charging voltage is increased to
60 V. The force-displacement curves shown earlier give an indication
that there would be proportionally less gain in velocity with a
higher charging voltage, although the gain should still be of the
order of 20 % (based on the work done). The actual peak velocity
using full shell enhancement and a 60 V charge is only a few percent
greater than using no enhancement at all.
There
are several possible reasons for this: the poor filling factor of
the shell material creates a distributed air gap which 'leaks' flux
and has a reduced permeability compared with solid iron; at higher
velocities the magnitude of the suckback impulse might become proportionally
larger thereby canceling much of the additional forward impulse
- the increased gradient about the midpoint of the force-displacement
curve for a flux-enhanced coil suggests that a flux enhanced configuration
could be more prone to suckback limitations as the muzzle velocity
is increased.
We
can also see from these figures that flux enhancement can increase
the muzzle velocity sensitivity to launch delta in the region around
-5 mm to 0 mm. This is expected since the simulated force-displacement
curves show that flux enhanced configurations produce a lower projectile
force in this region - if the projectile acceleration is reduced
at the start of launch then most of the current pulse can decay
before the projectile moves into the region of higher force, greatly
reducing the muzzle velocity.
Effect
of flux enhancement on efficiency. From fig 6a we can see
that low voltage peak efficiency is improved by an appreciable amount
when flux enhancement is employed. The efficiency gains at a charging
voltage of 60 V are minimal. Table 1 shows peak efficiency data
for both the 33,000 uF and 13,600 uF capacitors at a charging voltage
of 30 V and 50 V respectively.
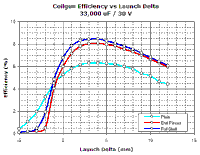
Fig
6a. Efficiency curves for various levels of enhancement
with a charging voltage of 30 V.
|
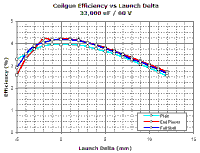
Fig
6b. Efficiency curves for various levels of enhancement
with a charging voltage of 60 V.
|
Capacitor |
Configuration |
Peak
Eff (%) |
Delta
(mm) |
Velocity
(m/s) |
|
Plain |
4.85 |
6 |
11.1 |
End
Pieces |
6.53
|
6 |
12.9 |
Full
Shell |
6.93
|
5 |
13.3 |
33,000
uF
|
Plain |
6.32
|
3 |
11.7 |
End
Pieces |
8.06
|
4 |
13.1 |
Full
Shell |
8.45 |
4 |
13.4 |
Table
1.
Extending
the parameter space for the 33,000 uF capacitor with full shell
enhancement yields the efficiency surface of figure 7. The efficiency
peak at a charging voltage of 15 V is just under 11 %, with the
velocity equal to 7.7 m/s.
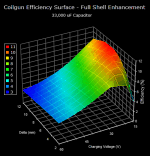
Fig
7. Voltage range extended down to 15 V giving an efficiency
peak of 10.9 %
|
Conclusions.
From the limited study presented here it is clear that flux enhancement
can deliver significant improvements in the velocity and efficiency
of a single-stage coilgun fired by an optically triggered MOSFET
switch. These gains are most evident when the coil current density
is low, as would be expected since the copper losses are low and
the permeability of the projectile and shell is high. There is little
difference between the velocity and efficiency gains offered by
end piece and full shell enhancement, so the additional expense
and effort involved in making a full shell doesn't seem to be justified,
at least in this particular case.
Taking
account of efficiency limiting factors such as the metallic accelerator
tube and low filling factor of the shell material it should be possible
to further improve efficiency. The effect of the current turn off
point in combination with diode commutation modification is another
avenue which can be explored.
Last
Modified: 30 Mar 2004
|