Electromagnetic Pistol:
CS-P01A
Voltage Converter Design
1
The
two most important considerations for this system are that the component
count and occupied volume should both be low. One system that fulfills
these requirements is the boost converter. A boost converter employs
an inductor, a switching device, and an output diode to generate
pulses of current at elevated voltage. The basic circuit is illustrated
in fig 1.
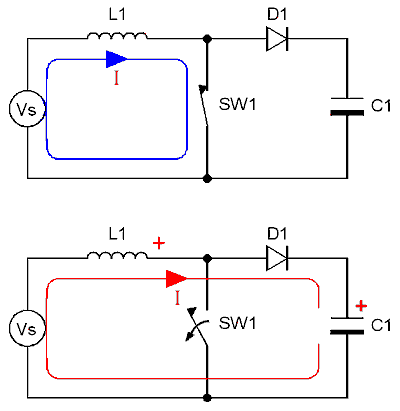
Fig
1. Principle of boost converter operation.
The
circuit operates as follows: When the switch is closed a current
is developed in the inductor which increases according to the time
constant of the circuit, this is termed the storage phase. The switch
is then opened after some small period of time and this causes the
voltage across the switch to increase as the inductor's collapsing
magnetic field attempts to maintain the current. Since the switch
effectively becomes an open circuit, the voltage continues to rise
until current is conducted through the output diode. Current is
driven through the diode until the energy that is stored in the
inductor is expended, this is termed the delivery phase. Since the
inductor can effectively boost the supply voltage by a factor of
10-15 it is possible to charge high voltage capacitors from a low
voltage battery source. The operating frequency of this type of
device can be anywhere between 50-250kHz so the diode and switch
must be capable of fast, efficient switching. A standard rectifier
diode and bipolar transistor would not be the ideal components in
this regard, although the circuit should still function at lower
frequencies. The
current waveform in the inductor will depend on the nature of the
load to which it is connected. A capacitor load behaves very much
like a constant voltage on the timescale of a storage/delivery cycle
so the current ramps down more or less linearly during each delivery
phase. As the capacitor voltage increases the delivery phase current
ramps down faster due to the larger inductor induced voltage. Note
that as well as the inductor's stored energy, the source (Vs) also
supplies energy during the deliver phase. This energy is the time
integral of the source power output during this phase, and it decreases
as the load voltage increases (the current ramps down faster so
the time that the source delivers power also decreases). Fig 2 illustrates
what the waveforms would look like.
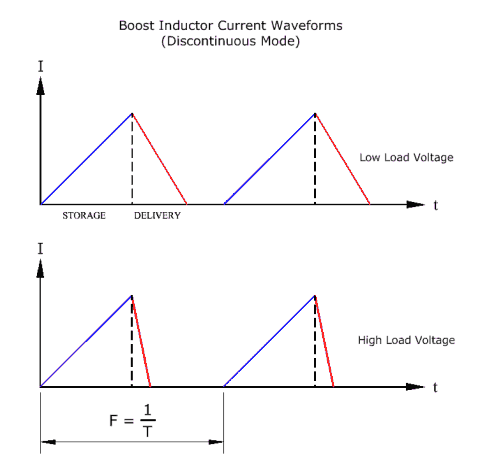
Fig
2. Typical current waveforms.
In
order to develop a useful embodiment of the boost converter, an
evaluation board was built in which the performance of test inductors
could be determined over various operating conditions. Fig 3 shows
the circuit board. Click on the image for a detailed diagram.
Fig
3. Boost converter evaluation board.
The circuit diagram
is given in fig 4. Click on the diagram for a larger version.
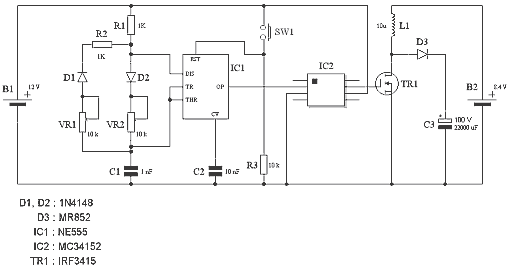
Fig
4. Boost converter evaluation circuit.
The
oscillator and MOSFET driver (IC2) are powered from the same 12
V source for the sake of convenience. While the oscillator could
run from 5 V upwards, the MOSFET driver really needs a 12 V supply
to ensure that it switches the MOSFET fully on during each cycle.
The mark and space time periods are independently variable by adjusting
VR2 and VR1 respectively. The typical operating frequency for this
circuit was 100 kHz. The oscillator is held in an off state until
SW1 is closed, it then operates until SW1 is opened again. The boost
converter section is powered from a separate 8.4 V @ 900 mAh NiCd
battery source.
Note
that the boost converter has the potential to continue charging
the capacitor until something breaks down. It's possible to add
an automatic gating facility to the oscillator such that the boost
converter stops charging when a preset voltage level is achieved.
This feature will be incorporated into the pistol control system.
The
time, T, taken to charge a capacitor to a specific voltage, Vc,
can be estimated from the following formula -
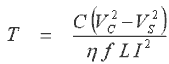
where
C is the capacitance, Vs is the supply voltage, n is the delivery
phase efficiency, f is the operating frequency, L is the inductance,
and I is the inductor current at switch turn off. The reason for
including Vs in the equation is that the capacitor initially charges
to this value through the diode when the supply is connected. Note
that this equation assumes that the inductor current starts and
ends at 0 during each cycle. This condition depends on both the
mark/space ratio and the output/input voltage ratio so the equation
is a rough guide. It also ignores the energy delivered by the source
during the delivery phase. This is reasonable if most of the charging
occurs with a large load voltage (say, Vload > 4Vs).
Something
else to consider is that a boost converter generates quite a lot
of EMI due to the rapid inductive switching (something which is
very apparent if you've got an AM station playing on a radio). It
is therefore necessary to pay attention to the layout of the circuit
board so as to avoid nasty noise coupling into the oscillator and
messing it up. There are ways of reducing the EMI which involve
controlling the turn off time of the MOSFET.
An
example of the charging behaviour is given in fig 4. Note the large
spikes present in the voltage waveform - a clear example of the
EMI being picked up by the 'scope.
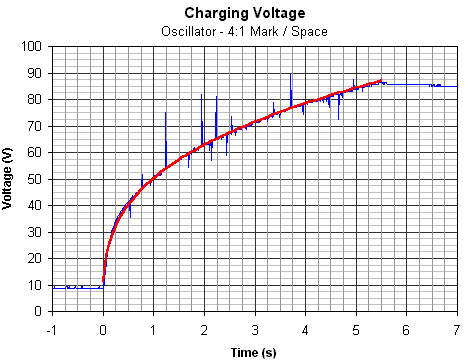
Fig
4. Charging voltage on stage 2/3 capacitors.
MORE
>
|